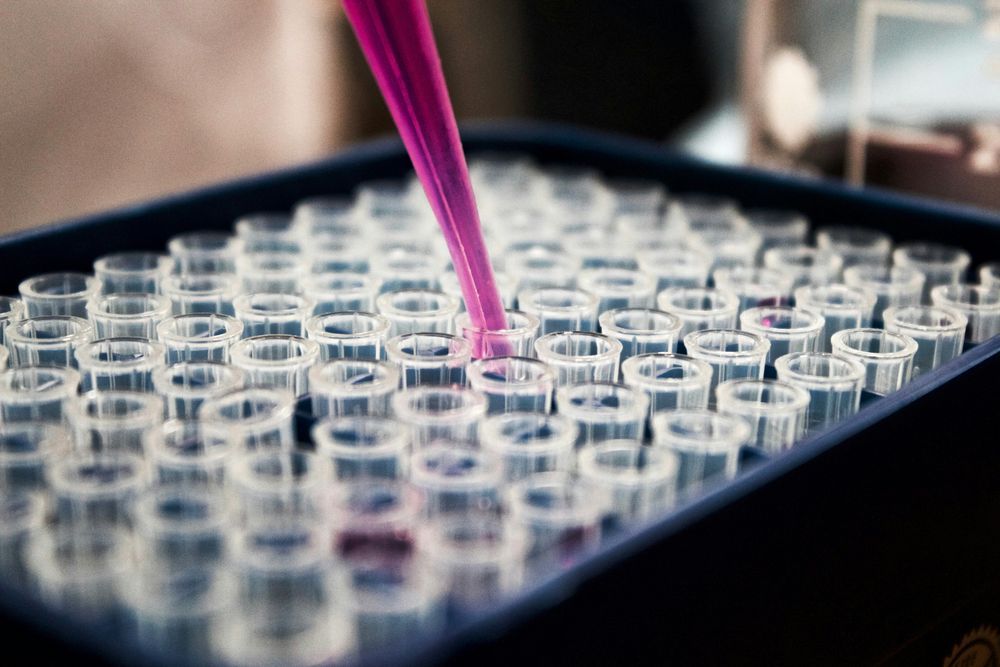
Glycosylation: Impact on Health and Disease
Learn about glycosylation, the complex process of attaching sugar molecules to proteins, and understand its influence on health and disease.
By Dora Lalić
The blueprint for how proteins are formed is encoded in our DNA, but that’s only part of the story. Once a protein is built, it can undergo further modifications through the attachment of various molecules, altering its structure and function. These changes, collectively known as post-translational modifications, play a crucial role in determining how proteins function in the body.
One of the most important modifications is glycosylation, where complex sugars are added to proteins. Over half of the proteins in the human body are actually glycoproteins, and they are involved in nearly every biological process.
There are two main types of glycosylation: N-linked and O-linked. In this article, we will explore O-linked glycosylation and its significance in human biology.
O-glycosylation is a process in which complex sugars, called glycans, are attached to the oxygen atom (O) of specific amino acids, typically serine or threonine, within proteins. This modification is essential for maintaining the stability, proper folding, and overall function of proteins.
Like all glycans, O-glycans exhibit remarkable structural diversity. They are composed of various smaller sugar units, including N-acetylgalactosamine (GalNAc), N-acetylglucosamine (GlcNAc), galactose (Gal), sialic acid, and fucose.
O-glycans can vary in length, ranging from short chains of 3-6 sugar units to longer, more extended structures. Typically, they are smaller and less complex than N-glycans, often forming biantennary (two-branched) structures.
O-glycans are found on a wide range of proteins, including cell surface proteins, secreted proteins, and mucins—specialized proteins that protect the respiratory and gastrointestinal tracts. Due to their widespread presence and diverse structures, O-glycans are involved in many essential biological processes, such as cellular signaling, immune system regulation, defense against pathogens, and reproductive functions.
Given their critical roles, it's unsurprising that abnormalities in O-glycosylation can lead to serious health issues. Altered O-glycosylation is frequently observed in various disease contexts and can arise from genetic mutations, as seen in congenital disorders of glycosylation, or from disruptions in the glycan biosynthetic machinery due to inflammation, infection, or cancer.
O-glycosylation occurs inside the Golgi apparatus. It begins with the addition of a single sugar called N-acetylgalactosamine (GalNAc) to the protein by an enzyme called GalNAc transferase.
The single sugar can further be modified by different enzymes that add additional sugars,, creating core structures that serve as the foundation for building complex O-glycans. There are 8 main core structures found in humans.
These core structures can be further modified by the addition of sugars such as Gal, GlcNAc, fucose, and sialic acids, giving rise to a diverse array of O-glycans.
The formed O-glycoproteins can then:
O-glycans are incredibly diverse and widespread, allowing them to serve many important roles in the body. They influence the proteins they are attached to by helping them fold correctly and function properly. Additionally, O-glycans interact with other molecules in the body to regulate various processes.
One key way O-glycans work is through a "lock and key" mechanism. Proteins called lectins are specifically designed to recognize and bind to particular O-glycans, similar to how a key fits into a lock. When this connection happens, it triggers a series of cellular reactions that can affect everything from cell communication to immune system responses.
O-glycans play a significant role in the interactions between pathogens and the host, particularly during infections. Bacteria often display O-glycans on their surface, which can mimic those of the host to evade immune detection and facilitate infection. This camouflage allows bacteria to blend in and avoid being targeted by the immune system.
O-glycans are essential for the immune system, especially in the maturation and activation of immune cells. When the body faces an infection or injury, immune cells like leukocytes are mobilized to the affected area. O-glycans, present on the surface of these cells, interact with lectins on blood vessel walls, helping the leukocytes roll along the vessels and guiding them more effectively to the site of damage where they are needed most.
O-glycans are vital for fertility, as they help manage the development of eggs in the ovaries. They also play a key role in the fertilization process—the outer layer of the egg, known as the zona pellucida, is covered with O-glycans that help sperm attach and fertilize the egg, making conception possible.
Mucins are large proteins that are abundant in O-glycans and are a key component of mucus, a protective barrier produced by various tissues throughout the body. They are primarily located on the surfaces of the respiratory, gastrointestinal, and reproductive tracts, where they form a protective and lubricating layer, but they can also be found on the surface of the eye and in the mouth.
Mucus plays a critical role in trapping and clearing pathogens, particles, and debris from these surfaces, while also providing a barrier that helps prevent infections and maintain tissue health.
There are two main types of glycosylation: O-linked and N-linked, which differ in where the glycan attaches to the protein. In N-linked glycosylation, the glycan attaches to the nitrogen atom (N) of the amino acid asparagine (Asn), but this attachment only occurs if asparagine is part of a specific sequence of amino acids - Asn/X/Ser/Thr, where X stands for any amino acids except proline.
In contrast, O-glycans can be attached to oxygen atoms of any serine or threonine residues within the protein.
Unlike O-glycosylation, which is a post-translational modification occurring after the protein has been synthesized, N-glycosylation starts during protein production, making it a co-translational modification.
This process starts in the endoplasmic reticulum (ER) where a pre-assembled sugar core is transferred to the protein from a lipid carrier. As the protein travels through ER and Golgi apparatus, it gets further modified by different enzymes, resulting in diverse and complex N-glycan structures.
In general, N-glycans are more complex than O-glycans. Both types of glycan share some common sugar building blocks, such as galactose, fucose, sialic acid, and N-acetylglucosamine (GlcNAc). However, some sugars are specific to one type of glycosylation.
For instance, mannose is a key component of N-glycans, forming part of their core structure, but it is rarely found in O-glycans. On the other hand, N-acetylgalactosamine (GalNAc) is predominantly associated with O-glycans and is not typically found in N-glycans.
Both N- and O-glycans play various important roles across all biological systems, and both types are integral for proper cellular communication, regulating immune responses, and supporting overall health.
Due to their crucial role in various biological systems, alterations in O-glycans are linked to a wide range of diseases, including genetic disorders, inflammatory conditions, and cancer.
Congenital disorders of glycosylation (CDGs) are a group of rare genetic diseases caused by defects in the glycan synthesis pathway. With over 100 subtypes identified, CDGs can impact multiple organ systems, leading to a wide range of symptoms.
These may include developmental delays, neurological issues, immune system dysfunction, and complications with the liver, heart, and other organs. The severity and specific symptoms vary depending on the particular subtype of CDG.
O-glycans play a crucial role in regulating the immune response, so abnormalities in O-glycosylation are linked to inflammatory conditions like inflammatory bowel disease (IBD).
As key components of mucins—the proteins that form protective barriers in the gastrointestinal tract—O-glycans help maintain the integrity of the mucus layer in the intestines. When O-glycosylation is disrupted, this protective barrier can weaken, leaving the epithelial cells vulnerable to microbial invasion and inflammation.
Alterations in O-glycosylation are seen in various cancers and play a critical role in cancer progression. These changes can promote tumor growth, invasion, and immune evasion. For instance, cancer cells can modify their O-glycans to avoid detection by the immune system, allowing them to grow unchecked.
Additionally, altered O-glycans can enhance the ability of cancer cells to migrate and invade other tissues, contributing to the spread of cancer, known as metastasis.
O-glycosylation plays a significant role in therapeutics, particularly in the development of biologic drugs and targeted therapies. Since O-glycans influence protein stability, folding, and function, they are critical in the design and production of therapeutic proteins, such as antibodies and enzymes.
In cancer therapy, understanding the specific patterns of O-glycosylation in tumor cells can lead to the development of novel biomarkers and targeted treatments. For example, therapies can be designed to inhibit the altered O-glycans that help cancer cells evade the immune system or to exploit these modifications to deliver drugs more effectively to cancerous tissues.
Additionally, O-glycosylation is essential in the design of vaccines and anti-viral therapies. By targeting specific O-glycans that pathogens use to infect host cells, therapeutic interventions can be developed to block these interactions, thereby preventing or treating infections.
Overall, O-glycosylation is a critical factor in the advancement of precision medicine, offering new avenues for the treatment of various diseases.
Both O- and N-glycans play essential roles in the human body by modulating protein activity and facilitating cellular communication. Their diverse functions and widespread presence make them key players in our health and disease, highlighting the importance of glycan research in advancing our understanding of various conditions and developing targeted therapeutic strategies.
Glycans also have remarkable potential as personalized health biomarkers. GlycanAge is a biological age test that measures chronic inflammation through N-glycans attached to antibodies.
This test provides insights into how your body is aging from within by reflecting the cumulative impact of genetics, epigenetics, and lifestyle. It also serves as an early warning system, as chronic inflammation drives disease development with age, offering you a chance for proactive health management before diseases develop.
You can learn more about GlycanAge by taking a short health quiz!
By Dora Lalić
Start or continue your GlycanAge journey
Don’t be afraid to reach out to us and ask questions, provide commentary or suggest topics.
Other articles you may like:
Learn about glycosylation, the complex process of attaching sugar molecules to proteins, and understand its influence on health and disease.
Discover all about glycation: its definition, harmful effects, contrast with glycosylation, impact on skin ageing, diabetes, heart health, and tips to reduce it.